Profiles in Diagnostics: Making Walls Better by Watching Walls Fall
Igor Bykov studies how plasmas interact with fusion reactor walls using high speed imaging and spectroscopy.
The tokamak approach to magnetic confinement fusion uses a toroidal solenoid to confine high-temperature plasmas. With peak plasma temperatures above 100 million degrees Celsius, no physical measurements are possible beyond the very edge of the devices. The unique diagnostic challenges of tokamak experiments are being overcome by talented international groups, including early-career researchers keen to develop fusion energy. Today’s profile follows the work of Dr. Igor Bykov as he uses advanced imaging techniques to investigate the ways in which interactions between the plasma and the device walls influence the behavior of the wider plasma.
Today’s international slate of tokamaks regularly produce plasma temperatures sufficient to cause nuclear fusion between deuterium and tritium, and what remains is to extend those operating regimes into steady-state power plant scenarios. An active area of fusion research deals with the large power fluxes that plasmas place on tokamak walls, which are capable of significantly surpassing 10 MW/m2. Particles of wall material can be ejected from the surface when bombarded with the energetic plasma particles. This process erodes the wall while also contaminating the plasma, leading to poor plasma performance. Because of this, extensive work is devoted to understanding the plasma-wall interactions as well as the optimal wall material for the affected areas.
Igor Bykov is advancing the physics that will elucidate how plasmas interact with tokamak walls and demonstrate the materials that are best suited for building future fusion power reactors. After completing his Ph.D. in 2015 from the KTH Royal Institute of Technology in Stockholm, Sweden, Bykov joined the University of California-San Diego Center for Energy Research (UCSD CER) and was stationed at the DIII-D National Fusion Facility to study plasma-wall interactions in tokamaks.
In a tokamak, plasma particles follow magnetic field lines that are carefully controlled to direct plasma exhaust into a divertor, which is a special region of the wall that is designed to absorb this power. The location where the magnetic field lines intersect with the divertor is called the strike point. Due to the ways in which the plasma itself can modify the total magnetic field, the strike point sometimes “splits,” which can direct particles into a wider area of the wall. Since this incident power flux leads to the aforementioned deterioration of the wall and plasma performance, it is important to understand which specific areas of the divertor plate need reinforcement to protect against these power fluxes. Typical, non-split, magnetic field lines leading to the divertor create two strike point locations that require reinforcement. In cases where appreciable strike point splitting occurs, however, there can be nearly a dozen separate strike points that deposit power well away from the reinforced divertor.
This situation is visualized in the following graphic. The left panel shows a typical magnetic boundary inside of which the plasma is confined. That boundary leads into the divertor and concentrates plasma exhaust power at the two labeled strike points. The right panel illustrates the more complex reality as calculated by a simulation that models all of the applied magnetic fields and the effects of the plasma on those fields. This Poincaré plot indicates the connection length of the field lines, which allows the strike point splitting to become starkly apparent. As a result of the splitting, there are now many more locations where significant plasma power is hitting the walls. The particular geometry of the DIII-D walls can lead to a condition in which some of this power spreading reaches weaker areas unintended for such incident power.
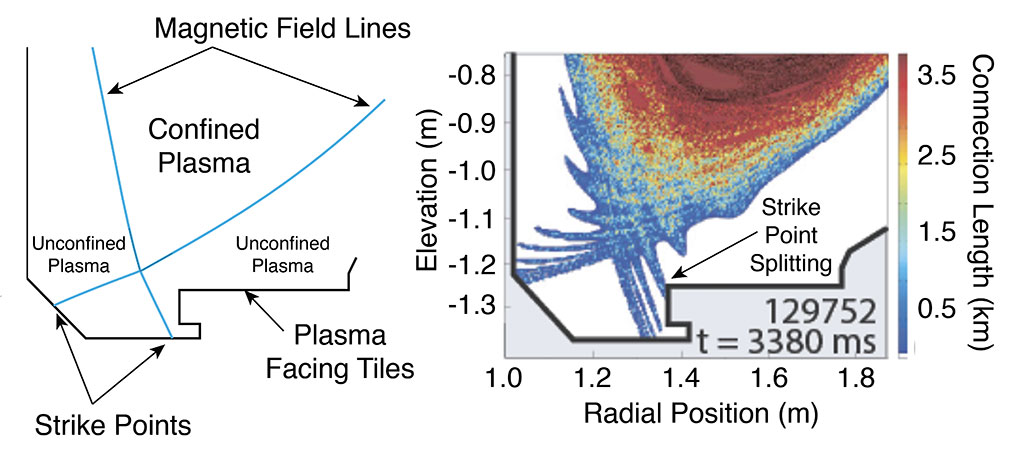
Bykov utilizes spectroscopy to identify these vulnerable points. His system relies on the visible emission of molecular deuterium, or D2 gas. These particles occur within the divertor tiles, and when the tiles are struck by the plasma, they release the molecular deuterium into the chamber. The heat from the plasma will immediately excite the molecular deuterium, which causes a photon to be emitted. Because this occurs within just millimeters from the tile surface (and only occurs where the tile is hit by the plasma), it provides a spatial localization that accurately tells us which part of the divertor plate we need to reinforce.
The tricky part, however, is distinguishing this emission from that of atomic deuterium, which is the atomic fuel that occurs in the plasma itself. The atomic deuterium can be excited and emit a photon within the plasma stream, even when it’s not close to the divertor wall. Molecular deuterium emits at a wavelength of 601 nm, while atomic deuterium emits at 656 nm. Bykov’s system is able to distinguish between these two close emission wavelengths. These measurements are particularly useful when acquired by high speed cameras that provide two–dimensional images of the various plasma-wall interactions (these Phantom cameras are commonly employed in imaging of combustion and other phenomena that occur on the timescale of 10-100 microseconds).
The figure below highlights the fidelity of this measurement. The left panel shows the lower divertor tiles, made of graphite, in DIII-D. Measured molecular deuterium emission is overlaid in yellow, and distinct bands are clearly identified. These are labeled as the Primary Inner Strike Point (ISP) and lobes. The inner strike point is closer to the center of the tokamak, while the outer strike point hits closer to outermost wall, i.e., largest major radius. A traditional imaging measurement of Balmer-alpha light at 656 nm is shown in the right panel, where the distinct divertor strikes, which are actually well localized on the tiles, appear as a more evenly blended mash between the X-point and inner strike point.
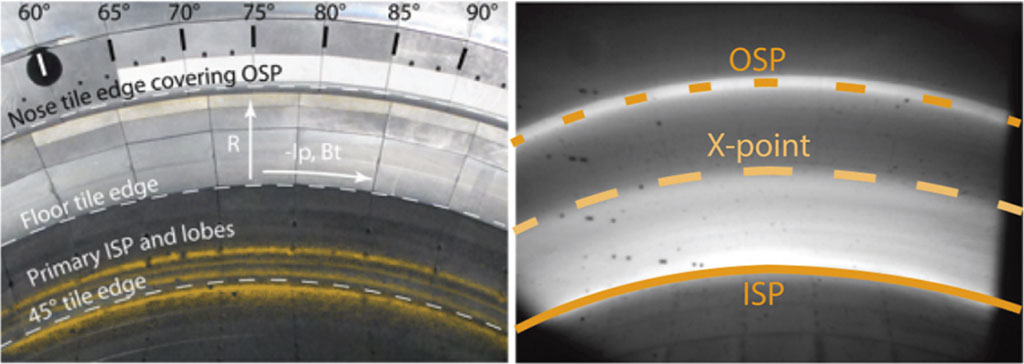
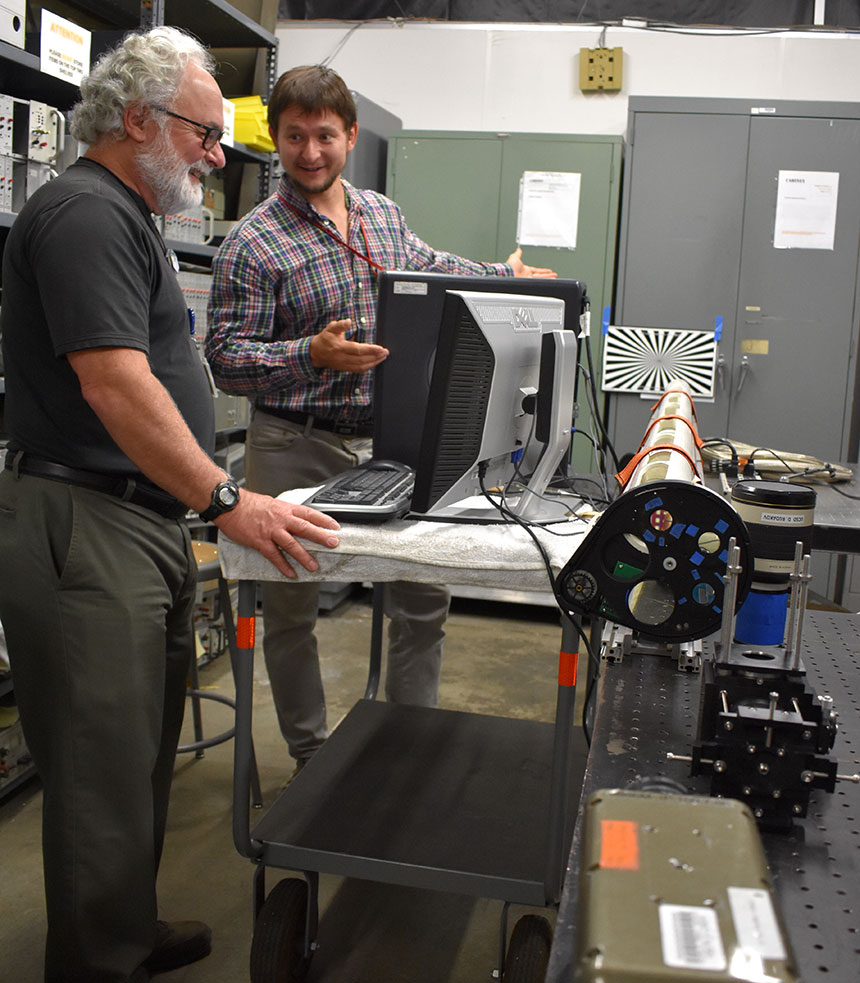
Rick Moyer, a Ph.D. plasma physicist within the UCSD CER, and co-recipient of the 2018 John Dawson Award for Excellence in Plasma Physics, discusses the importance of Igor’s work in the development of fusion research. “Understanding the ways deuterium ions interact at the plasma-wall boundary is crucial because deuterium is so important in the fusion reaction. When these ions come in contact with the wall of the tokamak, many things can happen: They can exchange charge with the wall (becoming neutral), they can recombine and form molecules, and they can be absorbed by the wall. This leads into why Igor’s work is so important for the next generation of fusion devices. At DIII-D, the tokamak walls are made of graphite, which absorbs and retains deuterium. However, the newer tokamaks have walls made of tungsten, which does not have this behavior. Igor’s work in spectroscopy gives us information that helps us understand how these plasma-wall processes differ from graphite to tungsten walls. In addition, by using the imaging of the same atomic and molecular processes at the divertor target, we are able to test and validate model predictions for how our 3D magnetic fields affect the plasma.”
The value of this diagnostic development extends beyond strike point splitting. Acquiring images of the plasma with kHz sampling rate informs experiments across a wide range of fusion research. Bykov’s system is in such high demand that the facility had to mark it as a “limited resource system” to authorize special resources that help keep it running safely and effectively. As the leader of the Advanced Materials Validation group within the DIII-D program, Tyler Abrams, Ph.D., appreciates the effort required to obtain these measurements. “Igor is an excellent spectroscopist. He has a really good intuition for how to build clever diagnostics to make very difficult measurements. His project involves discriminating between the atomic and molecular deuterium at a very high time and spatial resolution, which is extremely challenging. He is an extremely hard worker and is perhaps the first person to ever really successfully make these measurements.”
This work is supported in part by the U.S. Department of Energy, Office of Science, Office of Fusion Energy Sciences, using the DIII-D National Fusion Facility, a DOE Office of Science user facility, under Award DE-FC02-04ER54698, DE-FG02-07ER54917, and DE-FG02-05ER54809.
Additional Information:
U.S. Department of Energy, Office of Science, Office of Fusion Energy Sciences - https://science.energy.gov/fes/
DIII-D National Fusion Facility - https://fusion.gat.com/global/diii-d/home
ITER - https://www.iter.org/
UCSD Center for Energy Research - https://cer.ucsd.edu/
KTH Royal Institute - https://www.kth.se/en
Phantom High Speed Cameras - https://www.phantomhighspeed.com/
Rick Moyer’s Dawson Award citation - https://www.aps.org/programs/honors/prizes/prizerecipient.cfm?last_nm=Moyer&first_nm=Richard&year=2018
R.A. Moyer, I. Bykov, et al., “Imaging divertor strike point splitting in RMP ELM suppression experiments in the DIII-D tokamak,” Rev. Sci. Instrum. 89, 10E106 (2018)